Isoprenoids, also known as terpenoids, are a vast and diverse class of naturally occurring organic chemicals derived from five-carbon isoprene units. These compounds are crucial in various biological processes and have significant industrial and pharmaceutical applications.
Metabolic Pathways of Isoprenoids
Mevalonate Pathway (MVA)
The MVA pathway, prevalent in eukaryotes, archaea, and some bacteria, primarily operates in the cytoplasm of cells. It begins with the condensation of acetyl-CoA units and proceeds through several key steps:
1. Condensation of Acetyl-CoA:
- Acetyl-CoA Acetyltransferase (ACAT): This enzyme catalyzes the condensation of two acetyl-CoA molecules to form acetoacetyl-CoA.
- HMG-CoA Synthase (HMGS): Acetoacetyl-CoA condenses with another acetyl-CoA to produce 3-hydroxy-3-methylglutaryl-CoA (HMG-CoA).
2. Reduction of HMG-CoA:
- HMG-CoA Reductase (HMGR): HMG-CoA is reduced to mevalonate by HMGR, an NADPH-dependent enzyme. This step is the rate-limiting and most regulated in the MVA pathway, influenced by feedback inhibition, phosphorylation, and degradation mechanisms.
3. Phosphorylation of Mevalonate:
- Mevalonate Kinase (MVK): Mevalonate is phosphorylated to 5-phosphomevalonate.
- Phosphomevalonate Kinase (PMVK): The compound is further phosphorylated to 5-diphosphomevalonate.
4. Decarboxylation to Isopentenyl Pyrophosphate (IPP):
- Mevalonate Diphosphate Decarboxylase (MVD): The final step involves the decarboxylation of 5-diphosphomevalonate to IPP, with the release of CO2 and inorganic phosphate.
IPP serves as the fundamental five-carbon building block for isoprenoid biosynthesis. It can be isomerized to dimethylallyl pyrophosphate (DMAPP), another essential precursor.
Methylerythritol Phosphate Pathway (MEP)
The MEP pathway, also known as the non-mevalonate pathway, is found in most bacteria, algae, and plant plastids. It occurs in the plastidial compartment and involves the following steps:
1. Formation of 1-Deoxy-D-xylulose-5-phosphate (DXP):
- 1-Deoxy-D-xylulose-5-phosphate Synthase (DXS): Pyruvate and glyceraldehyde-3-phosphate (G3P) are condensed by DXS to form DXP.
2. Reduction to Methylerythritol Phosphate (MEP):
- 1-Deoxy-D-xylulose-5-phosphate Reductoisomerase (DXR): DXP is reduced and isomerized to MEP.
3. Formation of Cytidylated Intermediate:
- MEP Cytidylyltransferase (IspD): MEP is activated by adding a cytidine monophosphate (CMP) group to form 4-diphosphocytidyl-2-C-methyl-D-erythritol.
4. Phosphorylation and Cyclization:
- 4-Diphosphocytidyl-2-C-methyl-D-erythritol Kinase (IspE): This enzyme phosphorylates the intermediate to 4-diphosphocytidyl-2-C-methyl-D-erythritol 2-phosphate.
- 2-C-Methyl-D-erythritol 2,4-cyclodiphosphate Synthase (IspF): Cyclization occurs, producing 2-C-methyl-D-erythritol 2,4-cyclodiphosphate.
5. Reduction to HMBPP:
- 4-Hydroxy-3-methylbut-2-enyl Diphosphate Synthase (IspG): The cyclized product is reduced to 4-hydroxy-3-methylbut-2-enyl diphosphate (HMBPP).
6. Final Reduction to IPP and DMAPP:
- 4-Hydroxy-3-methylbut-2-enyl Diphosphate Reductase (IspH): HMBPP is reduced to IPP and DMAPP, which can then enter various biosynthetic pathways.
Integration and Regulation of Pathways
Though the MVA and MEP pathways operate in different cellular compartments and organisms, they are not entirely independent. Crosstalk between these pathways exists, allowing for metabolic flexibility. In plants, for example, IPP and DMAPP produced by the MEP pathway in plastids can be transported to the cytosol, where the MVA pathway operates, and vice versa.
Regulation of these pathways is complex and involves multiple layers of control, including:
- Feedback Inhibition: End products of the pathways, such as sterols and quinones, can inhibit key enzymes like HMGR and DXR.
- Gene Expression: Transcriptional regulation of pathway enzymes in response to environmental and developmental signals ensures the balanced production of isoprenoids.
- Post-Translational Modifications: Enzymatic activity can be modulated by phosphorylation, ubiquitination, and other modifications, providing rapid response mechanisms to cellular needs.
Downstream Biosynthetic Pathways
The basic five-carbon units, IPP and DMAPP, generated by the MVA and MEP pathways serve as precursors for a multitude of isoprenoid compounds through various downstream biosynthetic routes:
- Prenylation: Sequential condensation of IPP with DMAPP leads to the formation of longer-chain prenyl diphosphates like geranyl pyrophosphate (GPP), farnesyl pyrophosphate (FPP), and geranylgeranyl pyrophosphate (GGPP).
- Sterol Biosynthesis: FPP serves as a precursor for sterol biosynthesis, leading to the production of cholesterol in animals and phytosterols in plants.
- Quinone Biosynthesis: IPP and DMAPP are utilized in the synthesis of ubiquinones (coenzyme Q) and plastoquinones, essential electron carriers in cellular respiration and photosynthesis.
- Secondary Metabolites: Isoprenoids also contribute to the biosynthesis of a vast array of secondary metabolites, including terpenes, diterpenoids, and triterpenoids, which play roles in plant defense and signaling.
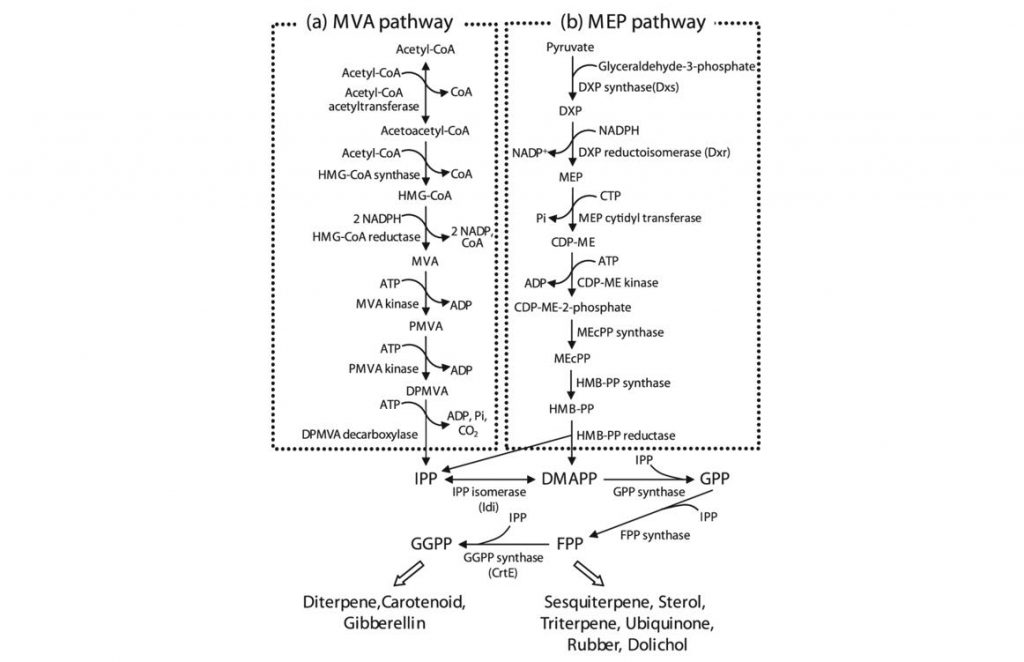
Isoprenoid biosynthetic pathway. Isoprenoids are synthesized via two independent early biosynthetic pathways: mevalonate (MVA) pathway (a) and 2-C-methyl-D-erythritol-4phosphate (MEP) pathway (b). (Harada et al., 2009)
Function of Isoprenoids
Roles in Plants
In plants, isoprenoids are crucial for several physiological processes and defense mechanisms:
Photosynthesis and Pigmentation:
- Chlorophylls: Isoprenoids contribute to the biosynthesis of chlorophylls, the green pigments essential for photosynthesis. Chlorophylls capture light energy and convert it into chemical energy, driving the photosynthetic process.
- Carotenoids: These pigments protect the photosynthetic apparatus by quenching reactive oxygen species generated during photosynthesis. They also contribute to the vivid colors of fruits and flowers, aiding in pollination and seed dispersal.
Growth Regulation:
- Gibberellins: These diterpenoid hormones regulate various aspects of plant growth and development, including seed germination, stem elongation, and flowering.
- Brassinosteroids: These steroidal hormones are essential for cell elongation, division, and differentiation, playing a vital role in overall plant growth and stress response.
Defense Mechanisms:
- Phytoalexins: Many isoprenoids act as phytoalexins, antimicrobial compounds synthesized in response to pathogen attack. They enhance the plant's defense system by inhibiting the growth of bacteria, fungi, and viruses.
- Volatile Organic Compounds (VOCs): Isoprenoids such as monoterpenes and sesquiterpenes are released as VOCs, which can repel herbivores or attract predators and parasitoids of herbivores, providing an indirect defense mechanism.
Roles in Animals
Steroid Hormone Synthesis:
- Cholesterol: Cholesterol is a primary isoprenoid and a precursor for steroid hormones, including cortisol, aldosterone, estrogen, testosterone, and progesterone. These hormones regulate metabolism, immune function, salt and water balance, and reproductive processes.
- Vitamin D: Synthesized from cholesterol, vitamin D is essential for calcium homeostasis and bone health.
Cell Membrane Structure and Function:
- Cholesterol: It is a vital component of cell membranes, contributing to membrane fluidity and integrity. Cholesterol helps maintain the proper function of membrane proteins and is involved in cell signaling pathways.
Coenzymes and Electron Carriers:
- Ubiquinone (Coenzyme Q): This isoprenoid is a critical component of the electron transport chain in mitochondria, playing a vital role in cellular respiration and ATP production.
Industrial and Pharmaceutical Applications
Drug Synthesis:
- Artemisinin: A sesquiterpene lactone used as a potent antimalarial drug, derived from the sweet wormwood plant (Artemisia annua).
- Taxol (Paclitaxel): A diterpenoid used as an anticancer agent, originally isolated from the Pacific yew tree (Taxus brevifolia).
Industrial Biotechnology:
- Biofuels: Isoprenoids like isoprene and farnesene are being explored as renewable biofuels due to their high energy content and compatibility with existing fuel infrastructure.
- Fragrances and Flavorings: Monoterpenes and sesquiterpenes are widely used in the production of essential oils, perfumes, and flavoring agents due to their aromatic properties.
Agricultural Applications:
- Pesticides: Natural isoprenoids like pyrethrins, derived from chrysanthemums, are used as insecticides due to their effectiveness and low toxicity to humans.
- Plant Growth Regulators: Synthetic isoprenoid analogs are used to enhance crop yields and stress resistance.
Techniques in Isoprenoid Analysis
Analyzing isoprenoids, with their vast structural diversity and critical biological functions, requires advanced and sophisticated techniques. At Creative Proteomics, we employ a comprehensive suite of methodologies to ensure precise and detailed analysis of isoprenoids. These techniques encompass various aspects of chromatography, mass spectrometry, and spectroscopic analysis, each contributing uniquely to understanding isoprenoid profiles, structures, and functions.
Chromatography is essential in the separation and quantification of isoprenoids due to their diverse chemical properties. Gas Chromatography (GC) is ideal for volatile isoprenoids and derivatives, such as monoterpenes and sesquiterpenes. GC offers high resolution and sensitivity, allowing the separation of complex mixtures. The procedure involves vaporizing samples and carrying them through a column with an inert gas, separating compounds based on volatility and interaction with the column material. High-Performance Liquid Chromatography (HPLC) is suitable for non-volatile and thermally labile isoprenoids, including carotenoids and steroids. HPLC provides versatility in column types and mobile phases, offering high separation efficiency. Samples are dissolved in a liquid solvent and pumped through a column, separating compounds based on polarity, size, and affinity to the stationary phase. Ultra-High-Performance Liquid Chromatography (UHPLC) enhances the resolution and speed of isoprenoid separation. It operates at much higher pressures than HPLC, enabling the use of smaller particle size columns for improved resolution and faster analysis times.
Mass spectrometry (MS) is pivotal for the detailed characterization and quantification of isoprenoids. Gas Chromatography-Mass Spectrometry (GC-MS) combines the separation power of GC with the detection sensitivity of MS. It is effective for identifying and quantifying volatile isoprenoids, providing structural information through fragmentation patterns. Liquid Chromatography-Mass Spectrometry (LC-MS) is suitable for a wide range of isoprenoids, particularly non-volatile and complex molecules. LC-MS offers high sensitivity and specificity, allowing for the detection of low-abundance isoprenoids in complex matrices. Tandem Mass Spectrometry (MS/MS) enhances structural elucidation and quantification of isoprenoids. Multiple stages of mass analysis and fragmentation provide in-depth structural details. In MS/MS, ions from the first MS are further fragmented and analyzed in a second MS, allowing for precise structural analysis and identification.
Spectroscopy provides additional structural and functional information about isoprenoids. Nuclear Magnetic Resonance (NMR) Spectroscopy is essential for detailed structural elucidation of isoprenoids. NMR offers non-destructive analysis, providing information on the molecular structure, conformation, and dynamics. Samples are subjected to a magnetic field, with resulting nuclear spin interactions offering insights into the molecular structure. Fourier Transform Infrared (FTIR) Spectroscopy is useful for identifying functional groups and characterizing isoprenoid structures. FTIR provides rapid and non-destructive analysis, offering information on molecular vibrations and functional groups. Infrared radiation is passed through the sample, and the resulting absorption spectrum is analyzed to identify molecular bonds and functional groups. Ultraviolet-Visible (UV-Vis) Spectroscopy is commonly used for analyzing conjugated isoprenoids such as carotenoids. UV-Vis provides information on the electronic structure and concentration of isoprenoids. Samples absorb UV or visible light, and the resulting absorption spectrum is measured, providing insights into the electronic transitions and concentration.
Advanced techniques and integrative approaches are often employed for comprehensive isoprenoid analysis. Isotope labeling and tracer studies are used to trace metabolic pathways and fluxes of isoprenoid biosynthesis. This approach provides insights into metabolic dynamics and pathway regulation. Isoprenoid precursors labeled with stable or radioactive isotopes are incorporated into metabolic studies, with subsequent analysis by MS or NMR to track their incorporation and transformation. Metabolomics and lipidomics involve broad-spectrum analysis of isoprenoid metabolites and their interactions with other metabolic pathways. This holistic approach provides a comprehensive view of isoprenoid metabolism and its integration with cellular processes. Comprehensive profiling of isoprenoid metabolites is performed using advanced LC-MS, GC-MS, and NMR techniques, combined with bioinformatics tools for data analysis and interpretation.
Reference
Harada, Hisashi, et al. "Efficient synthesis of functional isoprenoids from acetoacetate through metabolic pathway-engineered Escherichia coli." Applied microbiology and biotechnology 81 (2009): 915-925.