Carnitine, a quaternary ammonium compound, is an essential nutrient that plays a critical role in energy production by transporting long-chain fatty acids into the mitochondria, where they are oxidized for energy. It is also involved in the removal of toxic compounds from cells, preventing their accumulation. The biosynthesis of carnitine is a complex process that involves multiple enzymatic steps and is tightly regulated to ensure cellular energy homeostasis.
Chemical Structure of Carnitine
Molecular Composition and Structure
Carnitine's structure consists of a trimethylated quaternary ammonium head attached to a four-carbon backbone. This backbone includes a hydroxyl group (–OH) on the second carbon and a carboxylic acid group (–COOH) on the terminal carbon. The presence of the hydroxyl group classifies it as a hydroxy acid, specifically a β-hydroxy acid, which plays a significant role in its function as a carrier molecule for fatty acids.
Stereoisomerism: L-carnitine vs. D-carnitine
Carnitine exists in two stereoisomeric forms, L-carnitine and D-carnitine, due to the presence of an asymmetric carbon atom (the second carbon in the chain). The L-isomer is the biologically active form utilized by the human body, while the D-isomer is either inactive or can potentially inhibit the function of L-carnitine. The L-form is naturally occurring and is the one incorporated into enzymatic reactions and physiological processes.
Trimethylated Quaternary Ammonium Group
The quaternary ammonium group (N+(CH3)3) is a positively charged nitrogen atom bonded to three methyl groups. This group is essential for carnitine's solubility in water, allowing it to effectively transport across cell membranes and within different cellular compartments. The trimethylation of the ammonium group is a critical modification that occurs during the biosynthetic pathway, imparting carnitine with its unique properties.
Functional Implications of the Chemical Structure
The distinct chemical structure of carnitine is integral to its role in cellular metabolism. The hydrophilic quaternary ammonium group facilitates its interaction with membrane transporters, ensuring efficient uptake and distribution within the body. The hydroxyl group allows carnitine to form ester bonds with fatty acids, enabling the transport of these fatty acids into the mitochondria for β-oxidation.
Molecular Interactions and Stability
Carnitine's structure also contributes to its stability and interactions with other molecules. The zwitterionic nature of carnitine, with both positive and negative charges, allows it to maintain stability across a range of pH levels. This property is particularly important for its function in various cellular environments, from the acidic conditions in the stomach to the more neutral pH in the cytoplasm and mitochondria.
Synthetic and Natural Forms
While L-carnitine is naturally synthesized in the body, it can also be produced synthetically for use in dietary supplements and medical treatments. The synthetic process aims to replicate the exact structure of natural L-carnitine, ensuring its efficacy and safety. However, the bioavailability and absorption rates can vary between natural and synthetic sources, with naturally derived L-carnitine often being more efficiently utilized by the body.
Biosynthetic Pathway of Carnitine
The biosynthesis of carnitine is a multi-step process that converts the essential amino acid lysine into L-carnitine through a series of enzymatic reactions. This pathway primarily occurs in the liver and kidney and involves four key enzymes: Trimethyllysine Hydroxylase (TMLH), Hydroxytrimethyllysine Aldolase (HTMLA), Trimethylaminobutyraldehyde Dehydrogenase (TMABA-DH), and γ-Butyrobetaine Hydroxylase (BBOX).
1. Trimethyllysine Hydroxylase (TMLH)
The biosynthetic pathway begins with the methylation of lysine residues in proteins to form N6-trimethyllysine (TML). This modification typically occurs in proteins rich in lysine, such as histones or myosin. Once these proteins are degraded, free TML is released.
TMLH catalyzes the hydroxylation of TML to produce 3-hydroxy-N6-trimethyllysine (HTML). This reaction requires α-ketoglutarate, oxygen, and ascorbate (vitamin C) as cofactors and takes place in the mitochondria. The hydroxylation of TML is a crucial step, as it introduces a hydroxyl group into the molecule, enabling further processing.
2. Hydroxytrimethyllysine Aldolase (HTMLA)
The next step involves the cleavage of HTML by HTMLA, a cytosolic enzyme that splits the molecule into 4-trimethylaminobutyraldehyde (TMABA) and glycine. This aldol cleavage reaction is significant because it shortens the carbon chain of the molecule, preparing it for subsequent oxidation and conversion.
HTMLA's activity is vital for regulating the flow of intermediates in the pathway, ensuring that TMABA is produced at a rate compatible with its further oxidation.
3. Trimethylaminobutyraldehyde Dehydrogenase (TMABA-DH)
TMABA undergoes oxidation by TMABA-DH, an enzyme that converts it into γ-butyrobetaine (γ-BB). This dehydrogenase reaction occurs in the cytoplasm and requires NAD+ as a cofactor, which is reduced to NADH in the process. The oxidation of TMABA to γ-BB is a key step, as it forms the direct precursor to L-carnitine.
TMABA-DH not only plays a role in carnitine biosynthesis but also in the detoxification of aldehyde compounds, highlighting its importance in maintaining cellular health.
4. γ-Butyrobetaine Hydroxylase (BBOX)
The final step in carnitine biosynthesis is the hydroxylation of γ-BB by BBOX, an enzyme localized in the mitochondria. BBOX requires iron (Fe2+), α-ketoglutarate, and oxygen as cofactors to hydroxylate γ-BB, producing L-carnitine. This hydroxylation reaction is essential for converting the inactive γ-BB into the biologically active L-carnitine.
BBOX is tightly regulated and subject to feedback inhibition by L-carnitine, ensuring that carnitine synthesis is balanced with its physiological demand.
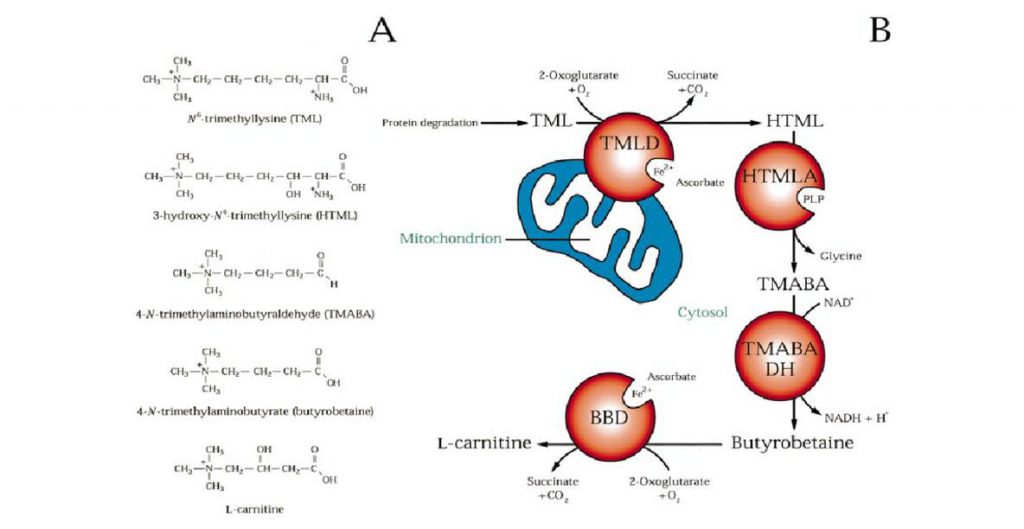
The carnitine biosynthesis pathway (Vaz et al., 2002)
(A) The chemical structures of the five carnitine biosynthesis metabolites. (B) Carnitine biosynthesis from TML. After release of TML by lysosomal protein degradation, this compound is hydroxylated
by TMLD, after which the resulting HTML is cleaved by a specific aldolase, which uses pyridoxal 5«-phosphate (PLP) as a cofactor, into TMABA and glycine
Substrate and Intermediate Compounds
Trimethyllysine (TML)
TML is the starting substrate for carnitine biosynthesis, derived from the methylation of lysine residues in proteins. The availability of TML depends on the protein turnover rate and the dietary intake of lysine.
Hydroxytrimethyllysine (HTML)
HTML is produced from TML by the action of TMLH. This hydroxylated intermediate is crucial for the subsequent cleavage and oxidation steps.
Trimethylaminobutyraldehyde (TMABA)
TMABA is generated from HTML through the action of HTMLA. As an aldehyde intermediate, it requires careful handling by the cell to prevent potential toxicity.
γ-Butyrobetaine (γ-BB)
γ-BB, formed by the oxidation of TMABA, is the immediate precursor to L-carnitine. The conversion of γ-BB to L-carnitine by BBOX is the final step in the biosynthetic pathway.
Regulation of the Biosynthetic Pathway
The carnitine biosynthetic pathway is tightly regulated to ensure homeostasis. This regulation occurs at multiple levels:
- Genetic Regulation: Genes encoding the enzymes of the carnitine biosynthetic pathway are subject to transcriptional regulation by factors responsive to metabolic needs.
- Nutritional Regulation: Adequate intake of lysine and vitamins (such as vitamin C and B6) is crucial for the optimal functioning of the biosynthetic pathway.
- Hormonal Regulation: Hormones like insulin can enhance the expression of enzymes involved in carnitine biosynthesis, adjusting the pathway’s activity in response to metabolic states.
- Feedback Inhibition: High levels of L-carnitine can inhibit the activity of BBOX, preventing excessive accumulation and ensuring balanced carnitine production.
Regulation of Carnitine Biosynthesis
The regulation of carnitine biosynthesis is multifaceted, involving genetic, nutritional, and hormonal factors. Tight regulation ensures that carnitine levels are maintained within a range that supports metabolic demands without causing toxicity.
Genetic Regulation
Genes encoding the enzymes involved in carnitine biosynthesis are regulated at the transcriptional level. Transcription factors responsive to cellular energy status can upregulate or downregulate the expression of these genes.
Nutritional Factors
Dietary intake of lysine and methionine, the precursors for carnitine synthesis, influences the biosynthetic capacity. Additionally, adequate levels of vitamins C, B6, and niacin are necessary cofactors for the enzymatic reactions.
Hormonal Influences
Hormones such as insulin and glucagon modulate carnitine biosynthesis by altering the activity of key enzymes. Insulin, for example, can enhance the expression of TMLH and BBOX, facilitating increased carnitine production during times of energy surplus.
Feedback Mechanisms
Carnitine levels are also regulated through feedback inhibition, where high levels of carnitine inhibit the activity of BBOX, preventing excessive accumulation.
Physiological Functions of Carnitine
Carnitine is indispensable for several physiological processes, primarily related to energy metabolism and cellular detoxification.
Fatty Acid Transport and Metabolism
Carnitine's most well-known function is its role in transporting long-chain fatty acids into the mitochondria for β-oxidation. This process is crucial for the production of ATP, especially during periods of increased energy demand such as exercise or fasting.
Energy Production
By facilitating fatty acid oxidation, carnitine helps maintain ATP levels, supporting various cellular functions. It also plays a role in stabilizing the acetyl-CoA/CoA ratio, which is vital for the tricarboxylic acid (TCA) cycle and energy production.
Detoxification
Carnitine assists in the removal of acyl groups from the mitochondria, preventing the accumulation of potentially toxic acyl-CoA compounds. This detoxification process is essential for cellular health and metabolic efficiency.
Immune System Support
Emerging research suggests that carnitine has immunomodulatory effects, potentially enhancing the function of immune cells and protecting against oxidative stress.
Disorders of Carnitine Biosynthesis
Defects in carnitine biosynthesis can lead to a range of metabolic disorders, often with severe clinical implications.
Genetic Defects and Their Impact
Mutations in the genes encoding carnitine biosynthesis enzymes can result in primary carnitine deficiency. This condition is characterized by low plasma carnitine levels and impaired fatty acid oxidation.
Symptoms and Clinical Presentations
Patients with carnitine biosynthesis disorders may exhibit symptoms such as muscle weakness, hypoglycemia, cardiomyopathy, and liver dysfunction. These symptoms arise due to the inability to adequately transport and metabolize fatty acids.
Diagnostic Methods
Diagnosis typically involves measuring plasma carnitine levels and identifying mutations in the relevant genes through genetic testing. Additional diagnostic tools include enzyme activity assays and metabolic profiling.
Treatment and Management Strategies
Management of carnitine biosynthesis disorders often involves carnitine supplementation to restore normal metabolic function. Dietary modifications and supportive therapies may also be necessary to manage symptoms and prevent complications.
Dietary Sources and Supplementation
While the body can synthesize carnitine, dietary intake contributes significantly to maintaining adequate levels.
Natural Sources of Carnitine
Carnitine is abundant in animal products such as meat, fish, poultry, and dairy. Plant-based sources contain significantly lower levels of carnitine, making supplementation important for individuals following vegetarian or vegan diets.
Bioavailability from Different Sources
The bioavailability of carnitine varies depending on the source. Animal-derived carnitine is generally more bioavailable than synthetic forms found in supplements.
Benefits and Risks of Supplementation
Carnitine supplementation can benefit individuals with metabolic disorders, athletes, and those with increased energy demands. However, excessive intake may lead to side effects such as gastrointestinal discomfort and potential cardiovascular risks.
Recommended Daily Intake
The recommended daily intake of carnitine varies based on age, sex, and physiological conditions. For most individuals, a balanced diet provides sufficient carnitine, but specific populations may require supplementation to meet their metabolic needs.
Reference
Vaz, Frédéric M., and Ronald JA Wanders. "Carnitine biosynthesis in mammals." Biochemical Journal 361.3 (2002): 417-429.